Mechanical quantum systems
Generating non-classical states of engineered macroscopic mechanical elements.
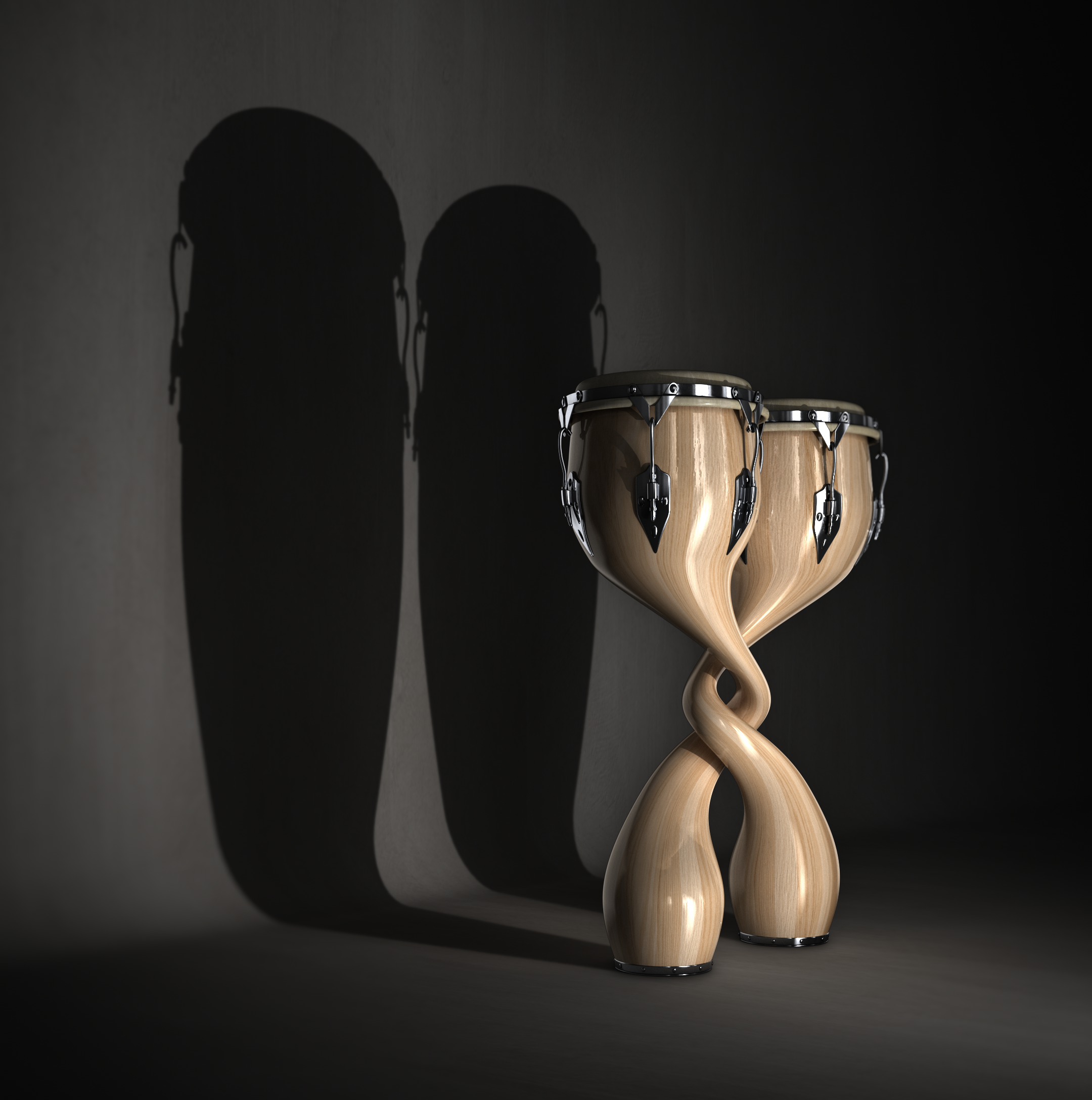
Previous results: Fabricated a superconducting resonator that couples to mechanical elements on chip. We use microwave signals to coherently control the mechanical elements at the quantum level. (1) Coupling a superconducting qubit to a mechanical element. A first step in generating non-classical states of a single mechanical element. (2) Coupling a mechanical element to a 3 dimensional bulk microwave cavity (Peterson et. al., “Ultrastrong Parametric Coupling between a Superconducting Cavity and a Mechanical Resonator”, PRL 123, 247701 (2019)). Reaching the ultra-strong coupling regime for the first time. (3) Building and measuring a novel architecture of two separate mechanical modes for full coherent control of mechanics. Generation of mechanical entanglement between two macroscopic mechanical objects. The chip made enables state preparation, coherent control of the two drums and simultaneous readout of the state of the two mechanical modes. It serves as a milestone on the path to a fully programmable multi-element mechanical processor. See Kotler et. al., “Tomography of Entangled Macroscopic Mechanical Objects”, Science 372, 622-625 (2021).
Quantum information theory
How to perform tomography of non-classical states, benchmarking of quantum processors, entanglement witnesses, continuous variables quantum mechanics, gravity induced constraints on quantum mechanics, the bootstrap method for error estimation, macroscopicity of quantum mechanics.
Hybrid quantum systems
Combining two separate degrees of freedom into a single quantum system in order to accommodate long memory time and fast processing speed in a single platform.
Previous results: Feasibility study of combining superconducting elements with trapped ions (Kotler et.al. “Hybrid quantum systems with trapped charged particles”, PRA 95, 022327 (2017)). We were able to rule out many architectures that were discussed in the community. On the converse, we showed that using electrons to carry the quantum information would enable processing speeds that are as fast as superconducting qubits (10s of nanoseconds) while retaining information as long as atomic systems (seconds).
Quantum sensing and coherent control
How to use quantum information techniques to enhance measurement sensitivity.
Previous results: A new technique to measure very small magnetic fields: the quantum lock-in (Kotler et.al. “Single ion quantum lock-in amplifier”, Nature 473, 61 (2011)). Our technique enabled us to extend the quantum memory time (coherence) of a single ion qubit by more than a factor of a 1000, from a millisecond to more than a second. Combining this technique and the use of ideas from Quantum Error Correction, we were able to measure the magnetic field of a single electron spin, at a distance of 2.4 um (Kotler et.al. “Measurement of the magnetic interaction between two bound electrons of two separate ions”, Nature 510, 376 (2014)). We were then able to use that measurement to place bounds on dipole-dipole couplings outside the standard model (Kotler et.al. “Constraints on Exotic Dipole-Dipole Couplings between Electrons at the Micrometer Scale”, PRL 115, 081801 (2015)).
Quantum measurement
What are the quantum limits of a measurement and how to attain that limit in superconducting quantum information processing experiments.
Previous results: Novel, non-reciprocal amplifiers that enable measurement of superconducting quantum bits with minimal back action i.e. minimal interference to the qubit being measured (Ranzani et.al. “Wideband Isolation by Frequency Conversion in a Josephson-Junction Transmission Line”, Phys. Rev. Applied 8, 054035, (2017), F.Lecoq et.al. “Microwave Measurement beyond the Quantum Limit with a Nonreciprocal Amplifier”, Phys. Rev. Applied 044005 (2020)). The main challenge here is measurement efficiency. Typically between 10%-20% it is limited by quantum mechanics in most architectures to 50%. In our architecture, we were able to surpass 50% with a clear path to get close to 100%. These works, not only enable state detection of the qubit, but also feedback steering of the qubit state to a desired state (quantum feedback).